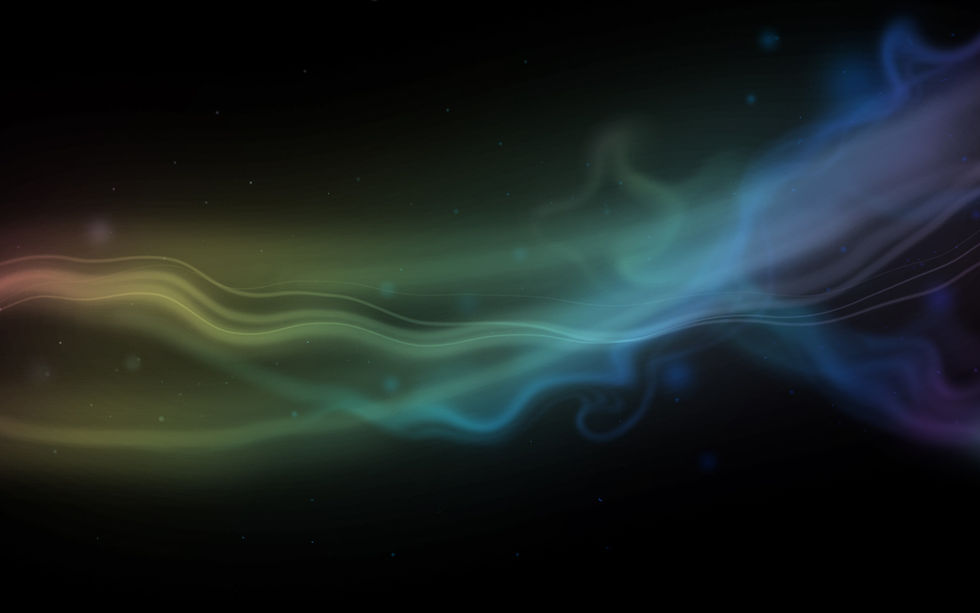
INERTIAL FUSION ENERGY
The fuel cycle for nuclear fusion involves extracting deuterium and lithium from sea water. Tritium is then ‘bred’ within the reactor from lithium. 1 litre of seawater could generate roughly 1 kWh of fusion power, meaning fusion can provide enough energy to power the world for millions of years. While this represents a huge technological challenge, it is clear that if a power production technology with sea water as the principal component could be made economically viable, there would be the potential for huge economic gains for the UK.
As a potential power source, our view is that laser fusion has huge potential, but, like all fusion schemes, faces significant technical challenges. Although a significant number of technical challenges are unique to a given fusion-approach, many challenges are shared between both magnetic and inertial fusion, as detailed in a recent paper. These shared challenges include tritium handling and the tritium cycle; materials and their survivability in the high-energy neutron environment of D-T fusion; neutronics and the validation of nuclear data; remote handling and maintenance activities; and integrated holistic approaches to fusion plant design. These highlight areas where knowledge-sharing and collaboration could be particularly beneficial between the inertial and magnetic fusion communities.
A significant number of projects have performed detailed design studies of how a laser fusion power plant might work in practice (e.g. HiPER and LIFE), while commercial fusion companies are actively investigating IFE reactor concepts.
In comparison to other fusion approaches Inertial Fusion Energy offers a number of potential technological advantages, as detailed in a comprehensive recent US National Academies of Science Engineering and Medicine report by the National Research Council. These are briefly discussed below.
A key potential advantage of the Inertial Fusion approach is the physical separation of the hot plasma from the surrounding infrastructure. For example, in laser fusion, the lasers can be focussed onto the target from a significant distance. This physical separation will reduce neutron and thermal damage to the surrounding infrastructure, potentially ameliorating significant technological risks associated with other fusion approaches.
Tritium is one of the two fuels required for fusion. Globally its supply is currently extremely limited. Research indicates that IFE power-plants have the potential to dramatically reduce the required Tritium inventory over other approaches.
IFE technology is inherently modular as the target physics is essentially decoupled from both the driver and power-plant technologies. This is advantageous as it enables progress to be made in parallel on all fronts: drivers, targets and physics. This decoupling also means that one scientific facility can be used to investigate numerous physics approaches to IFE in parallel.
Technological challenges unique to IFE include: the economical mass manufacture of targets; issues regarding the potential damage of final optics (in the case of Laser Fusion); the pulsed nature of any neutron and fast-ion damage; and target injection, tracking and survivability in the reaction chamber.
The National Academies report advocates promoting industry awareness of the complexity of the technology (a perceived barrier) and of the availability of complementary resources (a perceived advantage) for technology commercialisation by small and medium enterprises (SME). SME interest in forming bid consortia for a demonstration IFE fusion power plant and establishing the requisite supply chains would provide valuable leverage in realising IFE.
The indirect drive approach may well provide the initial demonstration of ignition; however, due to its inherent inefficiency in coupling laser energy to the implosion, it may not have sufficient fusion-energy-gain for practical power production. Furthermore, the hohlraum materials will cause the production of long-lived radioactive nuclides. Consequently, it is probable that either direct drive or a high-gain advanced scheme, as described in Section 8.1, will be required for power production purposes. This view was largely echoed during a Hooke discussion meeting on the “Prospects for High Gain Inertial Fusion Energy” at the Royal Society in London in March 2020, during which eighty international delegates met to discuss the state of the art and to discuss prospects for high fusion energy-gain.
With reference to the aforementioned National Academies report, the principle limiting factors in the economics of Inertial Fusion Energy are likely to be the cost of targets and, due to the potentially high capital cost, and the facility availability (limited by maintenance down-time). These characteristics imply that inertial fusion schemes which employ simple targets (that are amenable to economic mass-manufacturing) and have the potential for high fusion-energy-gain with relatively small driver energy-scale (thereby minimising capital cost), should be prioritised. In the specific context of laser fusion, these characteristics appear most compatible with advanced direct drive designs such as shock ignition, due to the simplicity and drive-efficiency afforded by excluding the hohlraum, and the potential for high-gain. We assert that the UK can best place itself to maximise scientific impact and take advantage of future economic benefits of inertial fusion energy by becoming global leaders in those areas of inertial fusion which have the potential for high fusion-energy-gain with low driver energy, and hence are most suitable for application to future power production.